What is the Difference Between a Wave Pulse and a Continuous Wave
Pulsed Wave Doppler
The pulsed wave Doppler (PW Doppler) sends short pulses of ultrasound and analyzes reflected sound waves between the pulses. This is accomplished by using the same piezoelectric crystals to send and analyze sound waves. The crystals alternate rapidly between sending and analyzing ultrasound. Therefore, emitted sound waves can be associated with reflected sound waves, making it possible to determine the distance of the reflector (i.e the structure reflecting the sound wave).
The pulsed wave Doppler can analyze sound waves reflected from a specific location. This is the main advantage of pulsed wave Doppler, namely its ability to determine the location of the measured velocities. However, the pulsed wave Doppler requires time to analyze reflected sound waves. This is due to the fact that the same piezoelectric elements are used to send and analyze sound waves. This reduces the maximum velocity that can be measured using pulsed wave Doppler. Generally, velocities above 1.5 m/s to 1.7 m/s cannot be measured correctly.
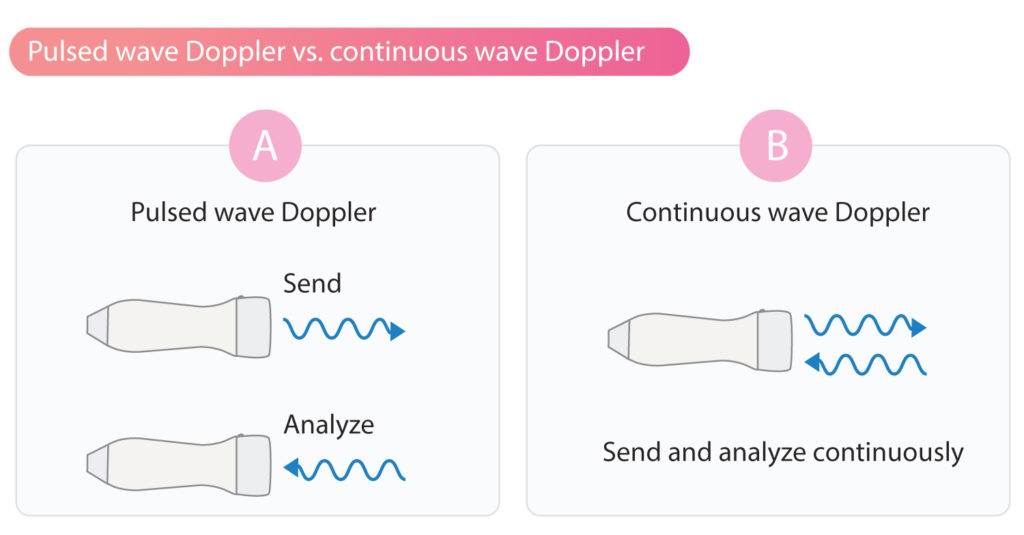
Sample volume (SV)
The major advantage of pulsed wave Doppler is the ability to specify where (along the Doppler line) to measure velocities. This is possible because the pulsed wave Doppler sends and analyses sound waves sequentially. The ultrasound machine is programmed to ignore all signals, except those reflected from a certain depth. The depth can be determined since the speed of ultrasound is constant in the body. The investigator specifies where the measurement should be performed by moving the sample volume (SV) along the Doppler line. The sample volume is depicted with two lines perpendicular to the Doppler line (Figure 2).
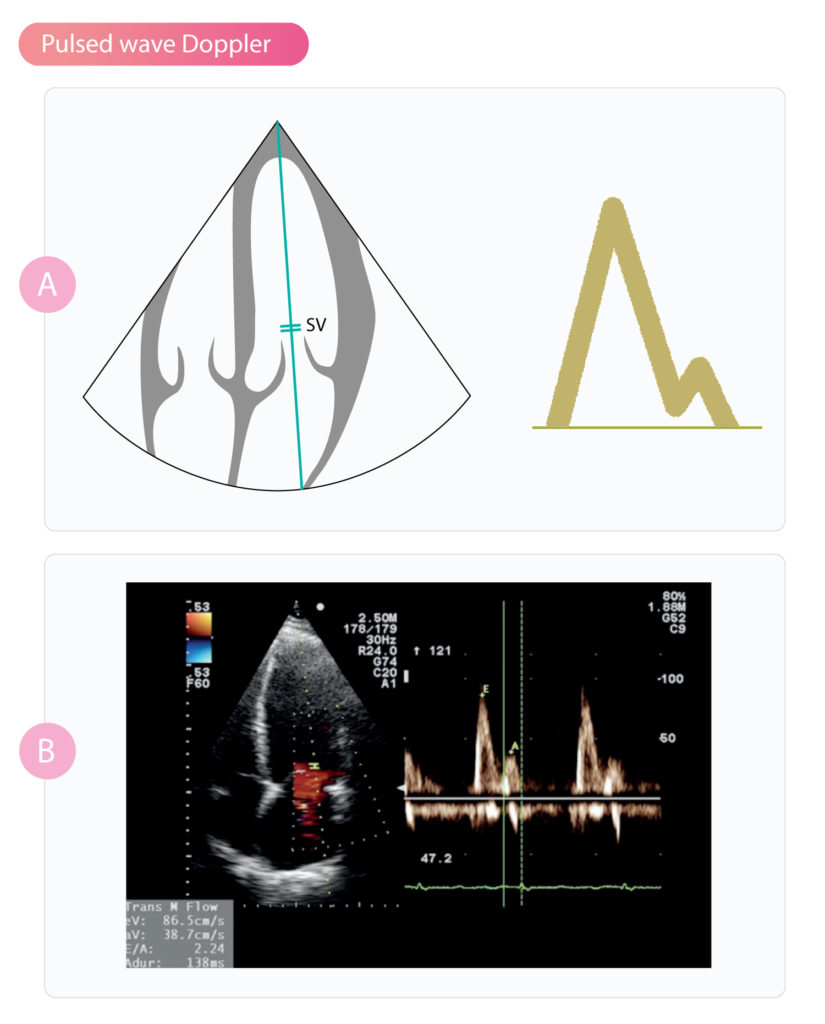
Pulse repetition frequency (PRF)
The number of ultrasound pulses sent per second is called pulse repetition frequency (PRF). PRF is determined by the speed of sound and the distance it must travel. Since the speed of sound in the human body is constant (1540 m/s), the PRF only depends on the distance the sound waves must travel. The longer the distance, the more time required for sound waves to travel back and forth, which results in lower pulse repetition frequency (fewer ultrasound pulses can be sent per second).
Pulse repetition frequency is inversely related to the distance the sound waves must travel. Visualizing distant structures result in lower pulse repetition frequency and consequently lower resolution. Visualizing proximally located structures enables the use of greater pulse repetition frequency, which results in greater resolution.
PRF must be high in order to assess the velocity and direction of blood flow, otherwise, the calculations will be uncertain. This is explained by the fact that each ultrasound pulse generates a snapshot of blood flow. The greater the number of snapshots per unit of time, the more accurate the description of blood flow. This is illustrated in Figure 3, which depicts a clock observed 5, 3 and 2 times during one cycle. As shown in Figure 3A, it is possible to determine with certainty the direction of the rotation with 5 observations per cycle. Using 3 observations per cycle, it is not possible to determine the direction of the rotation. With 2 observations per cycle, it appears that there is no rotation. This example illustrates the significance of high pulse repetition frequency in order to obtain accurate assessments of blood flow and myocardial movement.
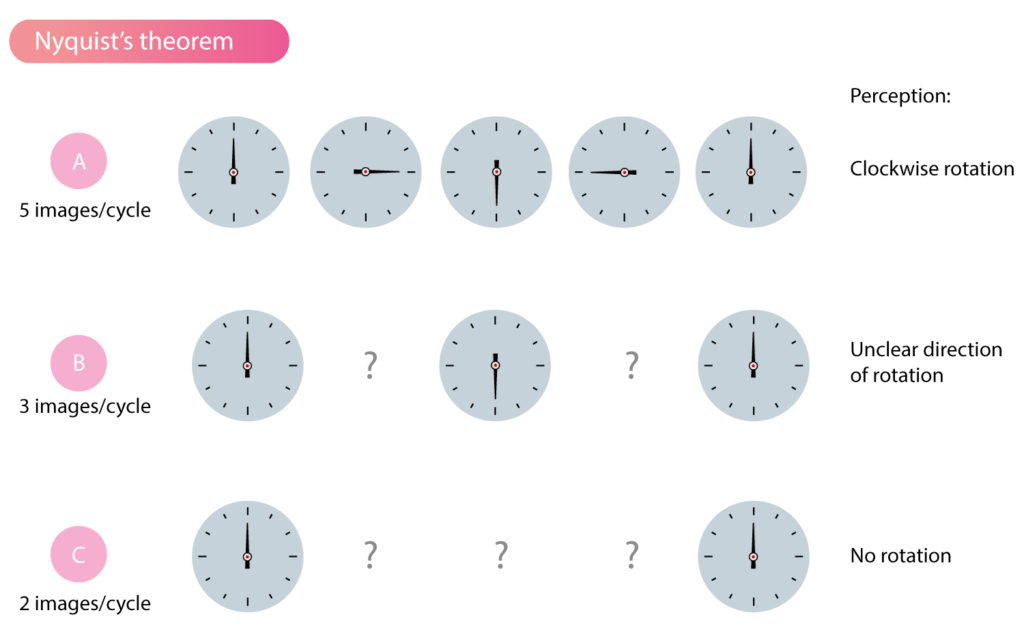
Nyquist's theorem and Nyquist limit
The importance of high PRF is explained mathematically by Nyquist's theorem (Harry Nyquist), which demonstrates that a wave must be sampled (i.e recorded) at least twice per cycle in order to be reliably measured. For pulsed wave Doppler, this implies that PRF must be at least twice the Doppler shift. Recall that the Doppler shift is directly related to the velocity of blood flow; the greater the velocity, the greater the Doppler shift. Thus, the maximum velocity that can be determined is half the PRF and this limit is called the Nyquist limit.
The maximum velocity that can be determined has a Doppler shift that is half the PRF. Hence, PRF must be at least twice the Doppler shift.
Aliasing phenomenon
Aliasing occurs if the velocity of blood flow exceeds the Nyquist limit. This implies that the ultrasound machine cannot determine the velocity and direction of the flow. On the ultrasound image, the velocities exceeding the Nyquist limit will be presented on the opposite side of the baseline. Positive velocities (i.e velocities normally shown above the baseline) exceeding the Nyquist limit will be shown as negative velocities and vice versa (Figure 4 and Figure 5).
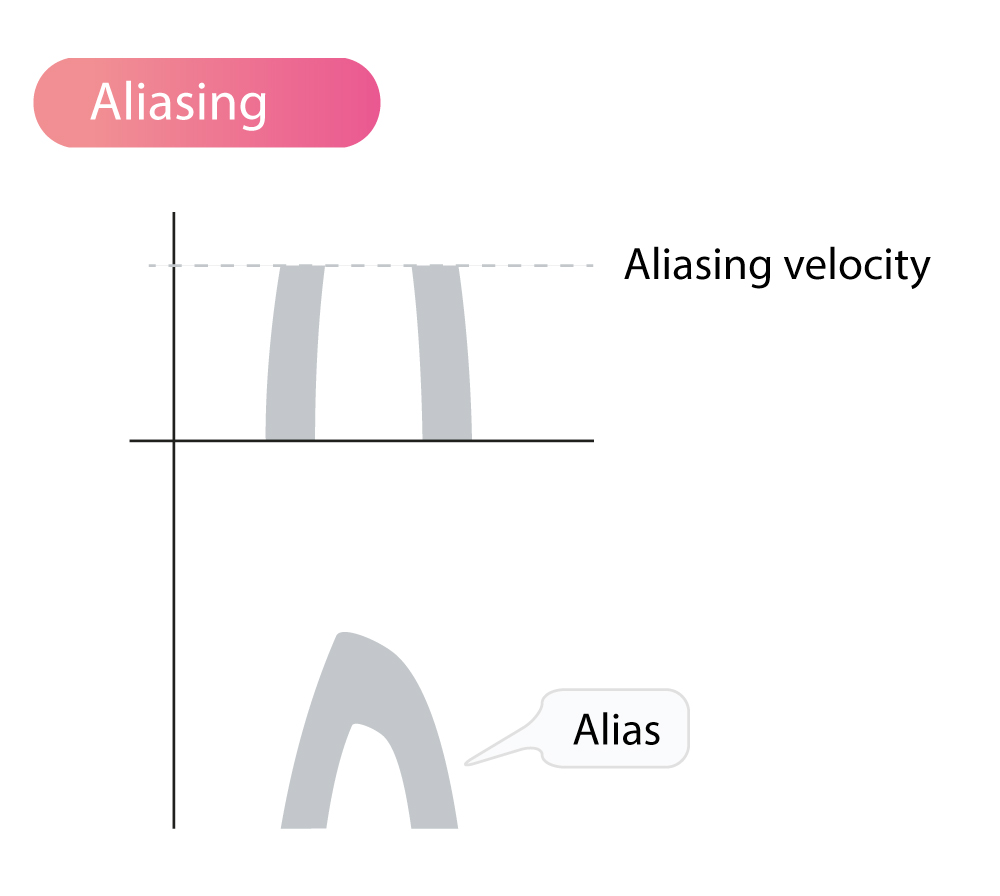
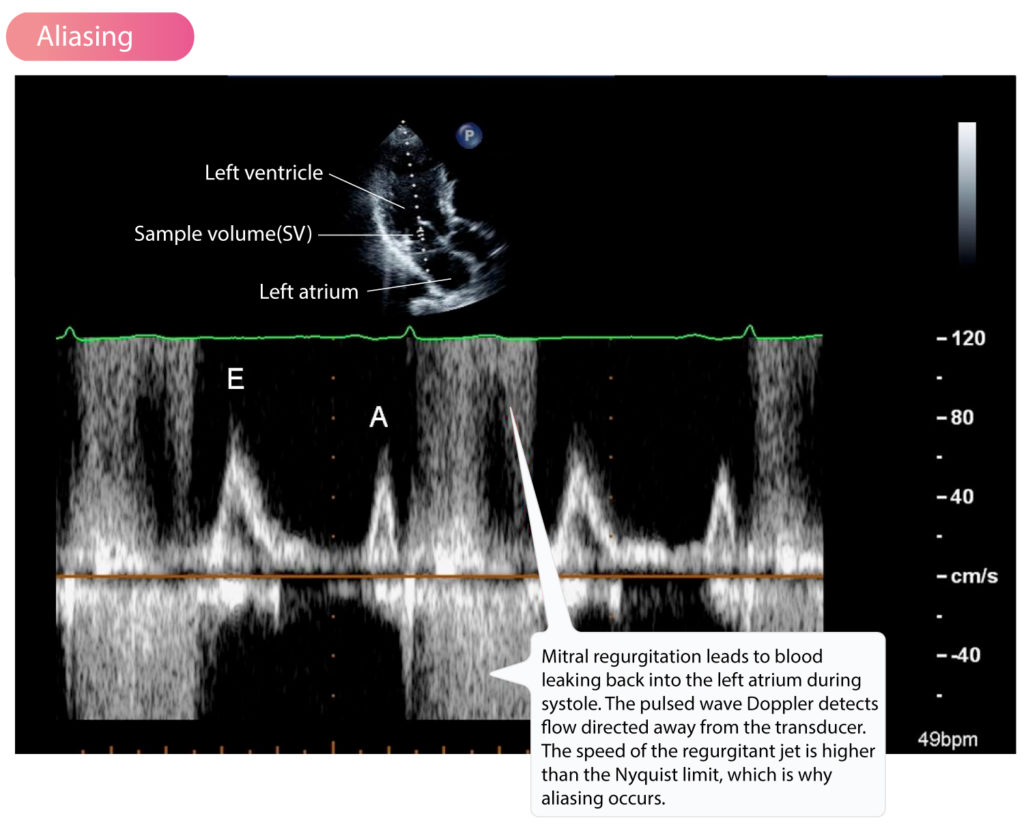
As mentioned above, the PRF depends on the depth being investigated. The depth is set by moving the sample volume along the Doppler line. The deeper the structures studied, the lower the PRF and, thus, the lower the maximum velocities that can be measured correctly, and vice versa.
Aliasing speed
It is straightforward to calculate the maximum velocity that can be measured using pulsed wave Doppler. Aliasing occurs when the velocity exceeds this maximum velocity (which is therefore referred to as the aliasing speed or aliasing velocity).
For example, at 15 cm depth, using ultrasound waves with a frequency of 3 MHz, the following equation calculates the time elapsed for sound waves to travel back and forth:
(0.15+0.15)/1540 = 0.0001948 seconds
Where 0.15 is the one-way distance in m; 1540 is the speed of sound (m/s) in the human body
The pulse repetition frequency (PRF) is calculated as the number of sound waves that can be transmitted and reflected per second:
PRF = 1/0.0001948 = 5133 soundwaves per second = 5133 Hz
The Nyquist limit (the maximum Doppler shift that can be detected) is half the PRF:
5133/2=2566 Hz
To calculate what flow velocity this corresponds, we use the Doppler equation:
v = (c·(fr-fe)) / (2·fe·cos ϴ)
We assume that the measurement is performed without any angle error, such that cos θ can be ignored. fe is the frequency of the emitted sound waves and fr is the frequency of the reflected sound waves. fr-fe equals the Doppler shift. c is the speed of sound (m/s) in the human body. The calculation follows:
v = (1540 · 2566) / (2 · 3000000) = 0.66 m/s
The maximum speed that can be measured is 0.66 m/s. If we increase the frequency of the emitted sound waves to 5 MHz, the maximum velocity that can be measured becomes:
v = (1540· 2566) / (2 ·5000000) = 0.40 m/s
It follows that we can reduce the frequency of the emitted sound waves to increase the aliasing speed; then aliasing occurs at higher velocities. It is also possible to adjust (by lowering or elevating) the baseline of the ultrasound image to reduce aliasing; doing this will adjust the PRF.
Aliasing can be remedied by reducing the frequency of the ultrasound or increasing the PRF.
Extended range doppler (High PRF Doppler)
Pulsed wave Doppler analyzes reflections from a specific location (i.e the sample volume) along the Doppler line. The maximum velocity that can be calculated is determined by the pulse repetition frequency (PRF), which is determined by the distance between the sample volume and the transducer. By using multiple sample volumes, the pulse repetition frequency is increased (the pulses from different sample volumes are added) and thus the aliasing speed is increased. This is referred to as high PRF Doppler or extended range Doppler. The advantage of high PRF Doppler is that greater velocities can be measured. Unfortunately, using high PRF Doppler makes it difficult to determine the location of the velocities recorded. To alleviate this problem, sample volumes are usually placed in areas known to have low flow velocities, making it possible to determine the location of higher velocities. Use of several sample volumes are illustrated in Figure 6.
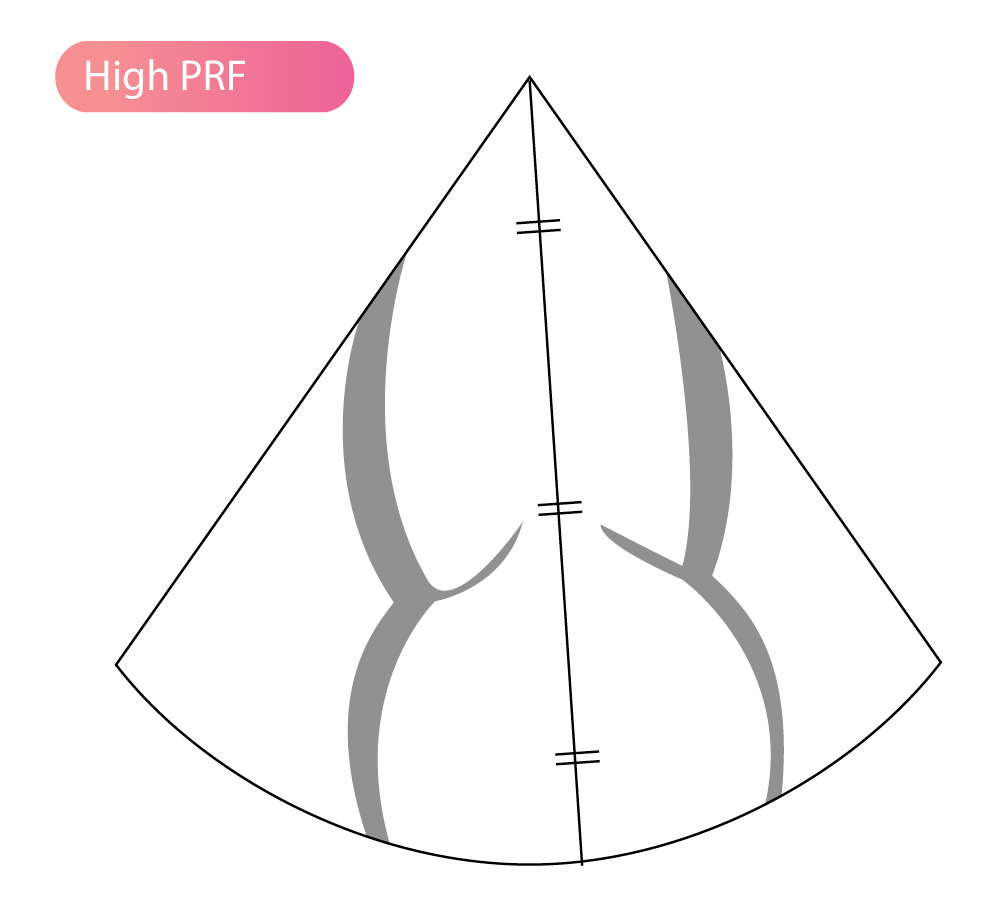
buntonjuserebeaven.blogspot.com
Source: https://ecgwaves.com/topic/pulsed-wave-doppler/